Hearing the Universe for the First Time
The Detection of Gravitational Waves and What That Has to Do With... Everything
February 15, 2016 | Revolution Newspaper | revcom.us
This past Thursday, February 11, came a very large, very cool, and very significant, announcement. Scientists found evidence of gravitational waves from a major event in the universe—a collision of two black holes 1.3 billion light years away from Earth. Albert Einstein predicted gravitational waves 100 years ago, part of his theory of how the universe works (the theory of general relativity), but it is only now that scientists have the instruments that can detect this. And his prediction is confirmed!
Working with Einstein's theory over time, it came to be understood by scientists to be basically true. It has led to many scientific breakthroughs, and it has continued to develop.
Successfully detecting gravitational waves is a very big deal—for science, and for humanity. Along with showing Einstein to be correct, it opens pathways to know much more, including now possibly being able to look farther back into the origins of the universe.
Communism is a science. And a great deal of the work of Bob Avakian, the Chairman of the Revolutionary Communist Party, USA, has focused on developing the scientific method of communism. He has emphasized that all actual truths discovered can help us understand reality more deeply, and can help us get to communism. Everyone can learn science.
We are including here a correspondence from a reader, videos and links to look into this. Correspondence on this is welcome. Write to revolution.reports@yahoo.com
Dig in, enjoy and learn!
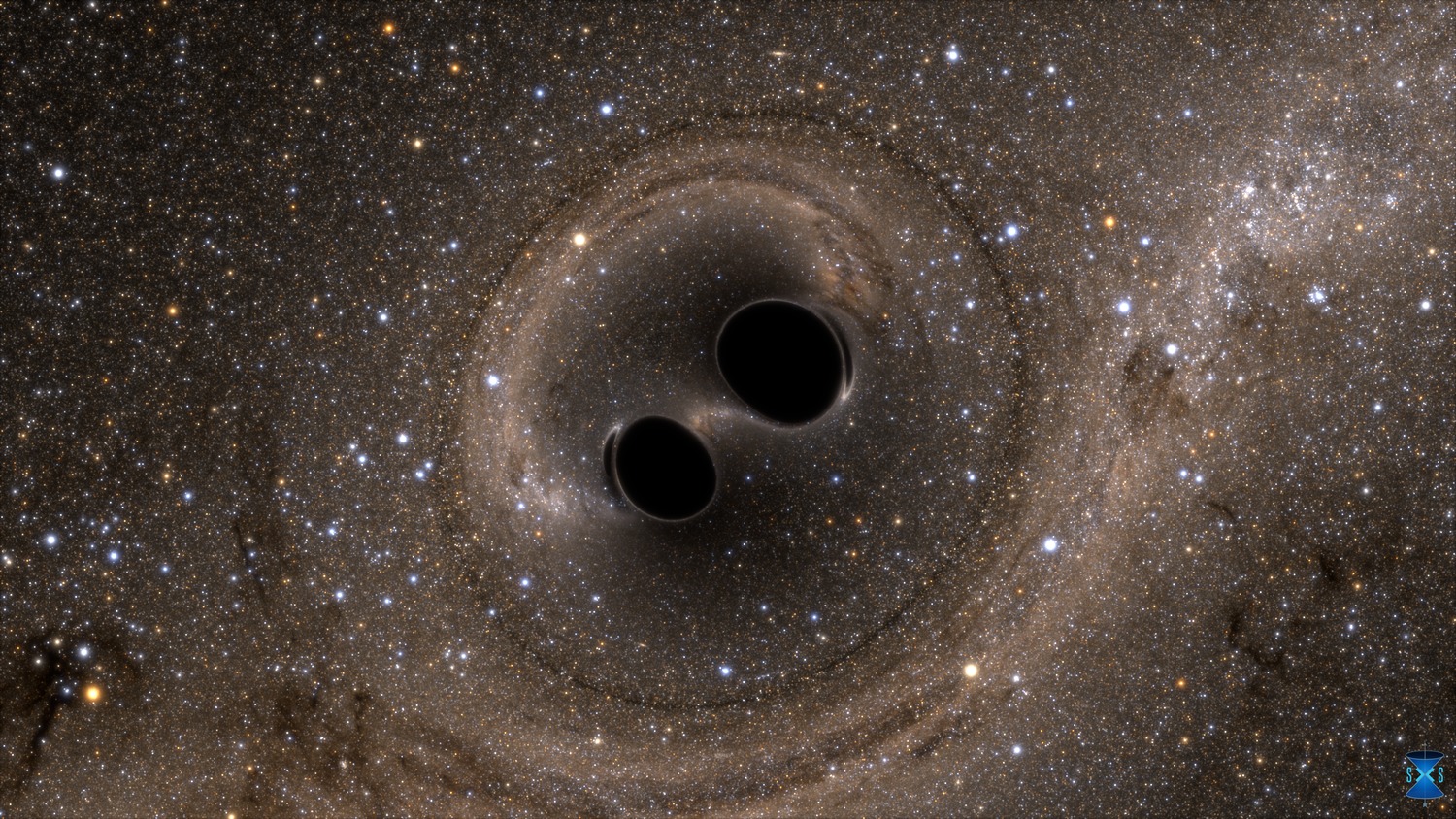
This still photo is from a video simulation showing how the collision of two black holes that were detected by the LIGO facilities might look if we could somehow travel in a spaceship for a closer look. It was created by solving equations from Einstein’s general theory of relativity using the LIGO data.
The stars appear warped due to the incredibly strong gravity of the black holes. The black holes warp space and time, and this causes light from the stars to curve around the black holes in a process called gravitational lensing. The ring around the black holes, known as an Einstein ring, arises from the light of all the stars in a small region behind the holes, where gravitational lensing has smeared their images into a ring.
This simulation was created by the multi-university SXS (Simulating eXtreme Spacetimes) project. For more information, visit www.black-holes.org
From a Reader:
Detecting Gravitational Waves
On Thursday, February 11, a worldwide team of scientists announced the first direct observation of gravitational waves—ripples in space-time caused by bodies in motion orbiting each other. This is a major scientific achievement. It directly confirms predictions of Albert Einstein’s general theory of relativity made a hundred years ago and opens up a whole new branch of astronomical investigation, a new window to understanding black holes and other massive objects in the universe, including even being able to “see” back way further into the history of our universe than has been possible—back to the time of the Big Bang itself.
Scientists collaborating on the Advanced Laser Interferometer Gravitational-Wave Observatory (LIGO) in the U.S. along with a European team called the Virgo Collaboration made the public announcement. This breakthrough is the culmination of decades of theoretical and practical work by an international network of scientists and engineers. On the same day of the announcement in the U.S., a report on the discovery was published in Physical Review Letters with over 1,000 authors from all over the world.
The gravitational waves were actually detected this past September by both of the two detectors of the LIGO project, first by the LIGO instruments in Louisiana and seven milliseconds (thousandths of a second) later by the instruments in Washington state. The observatories detected two massive black holes 1.3 billion light-years from Earth (one 36 times the mass of our sun and the other 29 times the mass of our sun) as they rapidly circled each other 30 times a second, accelerated to orbiting 250 times a second (at half the speed of light) and finally spiraled into each other forming a single black hole 62 times the mass of our sun—all in a period of just 20 thousandths of a second. During this instant of time an amount of energy equal to three times all the energy concentrated in the entire mass of our sun1 was transformed into gravitational energy causing a gravitational wave to be generated. If this energy were converted into energy as visible light it would be equivalent to the light of a billion trillion suns. Yet when it reached Earth, after spreading out from the collision for 1.3 billion years, the wave only moved the LIGO mirrors four thousandths of the diameter of a proton lasting for 20 milliseconds.2
On the LIGO instruments the passing of the gravitational wave came through as a brief chirping sound that could be analyzed in great detail by computers to determine if it was the signature of a gravitational wave as well as revealing detailed information about what caused the wave. The signal matched the predictions of general relativity for black holes in this situation precisely. Seven milliseconds after the instruments in Louisiana detected the wave, it was detected by the instruments in Washington, exactly as predicted by Einstein’s theory.
The LIGO observatories are not like telescopes we are familiar with. Each LIGO observatory consists of two very straight antennas which are tunnel-like arms of exactly the same length built horizontally along the ground, each 2.5 miles long and placed in an L-shape at exactly right angles to each other. These arms are heavily shielded from motion and disturbance, and inside is a very high (almost perfect) vacuum. Protected in this way are mirrors at the end of each arm hanging by thin glass threads. A laser beam is split at a point exactly the same distance from each of the mirrors and sent down the two tubes bouncing off of these two precisely positioned mirrors. Then the two beams are combined in a way that they can measure any tiny interference between them. Through precisely measuring any interference between the two beams, researchers are able to measure exceedingly small changes in the length of the arms and thereby measure the very, very tiny squeezing and stretching of space-time that is caused by any gravitational waves that might pass by. According to the theory of general relativity, the changes in distance caused by a gravitational wave will be extremely small. The LIGO instruments are able to detect a change in the length of the arms smaller then a ten-thousandth of the diameter of a proton. Put another way, this is equivalent to being able to measure a change in length over the distance from the sun to the next-nearest star—24.94 trillion miles away—to an accuracy of the width of a human hair.
The existence of gravitational waves is predicted in Einstein’s general theory of relativity, though at least early on, Einstein went back and forth in how sure he was of their existence. When he was convinced that gravitational waves existed, he said that the problems of actually detecting them were so great and the effects of them so subtle that he did not believe they would ever be found. For a hundred years there has been a quest to discover them. There have been 50 years of trial and error attempts to devise a way to detect them and at least 25 years of intense work to develop instruments sensitive enough for the job.
Through understanding Einstein’s theories, confirmation of other aspects of relativity theory, and through deepening and developing Einstein’s work, the overwhelming consensus among physicists and astronomers grew that gravitational waves existed as predicted. If it were proved that they did not exist, it would have been a major surprise and meant key and widely accepted theories about the universe would have to be scrapped. Then in 1974 astrophysicist Joseph Taylor and physicist Russell Hulse indirectly inferred the existence of gravitational waves by observing and tracking the motion of two massive and highly energetic super dense neutron stars rapidly and closely orbiting each other (one a pulsar emitting a periodic beam of light that could be timed as the two stars orbited each other). This was a big advance, but still there was no direct detection of an actual gravitational wave. Now that has all changed.
This new breakthrough has accomplished at least four major things: the first direct detection of gravitational waves; the first detection of a binary black hole (two black holes colliding and falling into each other); the best evidence to date that the nature of black holes is what is predicted by the general theory of relativity; and the opening up of a whole new branch of astronomy allowing far more detailed observations of black holes and other fundamental things about the universe.
The LIGO project has not only detected a gravitational wave, but also learned detailed information about the black hole collision that caused it. Despite just still being in its initial startup phase and despite being just the first of its kind to go into operation, it is already learning a tremendous amount. It only has only two detection arms and is working alone, making it difficult, for example, to more precisely determine where in the sky a gravitational wave comes from.3 Yet it was able to determine that the wave it detected came from the direction of the southern hemisphere and was 1.3 billion light-years away. Other observatories like the Virgo interferometer in Italy are in the works and LIGO is set to work closely with them to combine their observational power. Further, so far LIGO is only operating at one third of its potential. When it is fully operational it will be able to detect gravitational waves coming from 27 times the area of the cosmos that it now can observe.
Up until now observations of the universe have been through looking at light signals (this includes forms of light such as radio waves, microwaves, x-rays, gamma rays etc.). Now there is a whole new way to “see.” Observing gravitational waves allows us to see some things much more clearly and cleanly than by looking at light which is affected by the Earth’s atmosphere and other things. Perhaps most exciting is that until now there was a limit to how far back we were able to see to earlier times in the universe (the farther out we look, the farther back in time we are looking because of how long it takes the light to reach us). Until now there was a barrier at about 380,000 years after the Big Bang (which happened about 13.7 billion years ago) when everything is in principle opaque if we only have light to look at.4 But gravitational waves make transparent what has been blocking our view. So now we can potentially learn much more about what happened when our universe began.
“LIGO has opened a new window onto the Universe.”
—Kip Thorne, LIGO co-founder
“Until this moment we had our eyes in the sky and we couldn’t hear the music.”
—Szabolcs Marka, member of the LIGO team
“For almost all of humanity’s existence, the sole way in which we have studied our Universe is through light. This discovery has given us a new “sense” to explore the Universe, in a way opening our ears and allowing us to listen to the cosmos for the first time. Gravitational waves will allow us to probe objects, events, and epochs that are inaccessible to light, such as the merging of two black holes or the first second after the Big Bang... Needless to say, this detection will prove to be one of the most significant discoveries in modern physics, as it has opened an entire new realm of the Universe to explore.”
—Michael Zevin, graduate student and member of the LIGO team
Footnotes:
1 For an idea of how much energy this is: If just one gram of mass is converted entirely into energy it equals the amount of energy released by igniting 568,000 gallons of gasoline. The mass of the Earth is about 6,000,000,000,000,000,000,000,000,000 grams. The mass of the sun is 333,946 times the mass of the entire Earth. [back]
2 Since gravity is a very, very weak force in comparison to the other fundamental forces in nature, it takes huge masses—like black holes and neutron stars—moving close to each other to generate gravitational waves that are detectable by instruments that can be built by humans. In reality all objects moving around each other will cause ripples in the fabric of space-time resulting in carrying away energy, but this is an infinitesimally small effect for ordinary objects. To take one example, the strongest ripples in space-time in our solar system are caused by the orbit of the largest planet Jupiter around the sun. This motion only results in an energy loss to gravitational energy equal to the energy emitted by a 40-watt light bulb. [back]
3 Once the source of gravitational waves can be precisely determined it will then be possible to train Earth- and space-based conventional telescopes on the source to observe what is happening that they can see. This will yield even more information about what is going on at the sources of the gravitational waves. [back]
4 Before about 380,000 years after the Big Bang all the photons (fundamental particles or wave-packets of light) in the universe were bound up and trapped in interactions with the particles that would eventually form atoms—protons (positively charged particles in the atomic nucleus) and electrons (negatively charged particles orbiting the nucleus in clouds or “energy states”). These particles themselves could not have existed until the universe had cooled down from the Big Bang, and further cooling would have to happen before there was a possibility for atoms to form. As long as the universe was too hot, photons could not escape continuously interacting with these subatomic particles. Because of this, photons could not travel outside of the region of space that was filled with the protons and electrons. So it is not possible for us to see or detect anything via any form of light coming from a time before this ceased to be the case. After about 380,000 years after the Big Bang, protons and electrons mostly combined into hydrogen and helium atoms and the photons were free to travel as they will at the speed of light in all directions. The universe became transparent. We can detect these photons today as the cosmic microwave background radiation. From studying the cosmic microwave background radiation, a lot has been learned about the early universe, but there has been this limit going back to about 380,000 years after the Big Bang. [back]
People look at what religion calls “the heavens.” They look at the stars, the galaxies. They can see a small part of the vastness of the universe, and they can imagine the greater vastness of the universe. Or they can look on a small scale, look with a microscope and see a small microbe or whatever, and be amazed by what goes on internally within that. They can ponder the relation between what you can see with a microscope and what you can see with a telescope. This is an essential quality of human beings. Human beings will always strive for this. Far from trying to suppress this, or failing to recognize it, we can and should and will give much fuller expression to it.
Communism will not put an end to—nor somehow involve the suppression of—awe and wonder, the imagination, and “the need to be amazed.” On the contrary, it will give much greater, and increasing, scope to this. It will give flight on a much grander scale to the imagination, in dialectical relation with—and in an overall sense as a part of—a systematic and comprehensive scientific outlook and method for comprehending and transforming reality.
Bob Avakian, BAsics 4:30
Photo: www.ligo.caltech.edu
These graphs show the signals of gravitational waves detected by the twin LIGO observatories. The signals came from two merging black holes, each about 1.3 billion light-years away.
The top two plots show data received at the two facilities, along with the predicted shapes for the waveform. These predicted waveforms show what two merging black holes should look like according to Einstein’s general theory of relativity, along with the instrument’s ever-present noise. Time is plotted on the X-axis and strain on the Y-axis. Strain represents the fractional amount by which distances are distorted.
As the plots reveal, the LIGO data very closely match Einstein’s predictions.
The final plot compares data from both detectors. The Hanford data have been inverted for comparison, due to the differences in orientation of the detectors at the two sites. The data were also shifted to correct for the travel time of the gravitational-wave signals between the two facilities. As the plot demonstrates, both detectors witnessed the same event, confirming the detection.
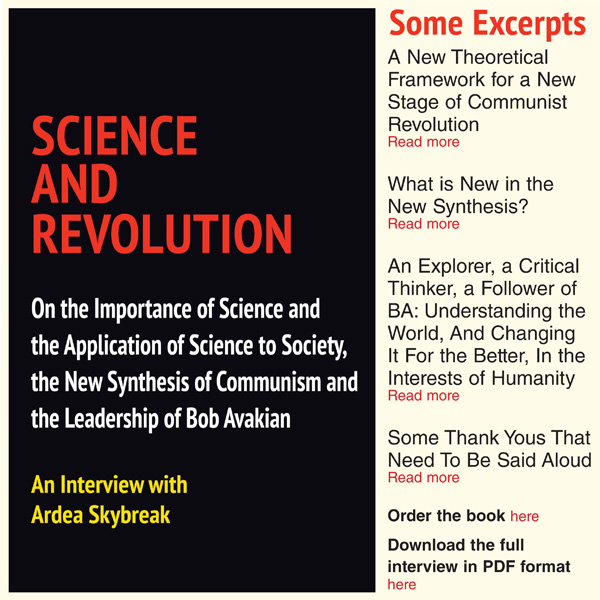
Volunteers Needed... for revcom.us and Revolution
If you like this article, subscribe, donate to and sustain Revolution newspaper.